The tumor microenvironment (TME) is a complex network of cells, molecules, and extracellular matrix that surrounds and interacts with cancer cells. It plays an essential role in the growth and progression of cancer. Understanding the cancer microenvironment is essential for developing new, more effective cancer therapies. As the usual two-dimensional (2D) cell culture model is poorly reliable, many efforts are made to find an appropriate model that faithfully reproduces the complexity of the cancer microenvironment.
In the last decades, with the improvement made in the field of tissue engineering, started to appear 3D cell culture solutions such as spheroid and cancer-on-chip. However, these solutions cannot still control the organization of multiple cell types in a complex dynamic 3D architecture.
3D bioprinting involves the deposition of bioinks layer-by-layer, spatially defined by computer-aided designed structure. Up to now, three main types of bioprinting exist: extrusion-based bioprinting (scaffold-free or scaffold-based), laser-based bioprinting, and droplet-based bioprinting. By allowing the mediation of spatial control of the matrix properties (like matrix stiffness, the effect of the cancer environment on cell migration, and spatial distribution of biomechanical factors), and having the ability to integrate perfusable vasculature into 3D architecture - allowing the study of the invasion of cancer cells through vessels - 3D bioprinting seems to be the better candidate to recapitulate the cancer microenvironment complexity (1).
3D bioprinting to reconstitute breast cancer TME
Breast cancer is one of the most common cancers that concerns around 2 million people worldwide. Breast cancer’s TME is characterized by heterogeneous cell types, varied extracellular matrix composition, mechanical properties of extracellular matrix (ECM), and cytokines and growth factors, which standard drug screening in 2D cell cultures fails to recapitulate (2).
In a study published by Zhou and colleagues, the researchers used 3D bioprinting to model interactions between breast cancer cells and bone stromal cells. Scaffolds were developed using stereolithography technology and gelatin methacrylate hydrogel with nanocrystalline hydroxyapatite (nHA). In this study, mesenchymal stem cells (MSCs) or human osteoblasts-laden bioinks were printed to develop the cell-laden scaffold and co-culture it with breast cancer cells. As a result of the coculture on the scaffold, they observed a rise in VEGF secretion and enhanced growth of the breast cancer cells while inhibiting the proliferation of MSCs/osteoblasts. (Figure 1) (3)
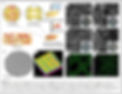
FIGURE 1 | An example of breast cancer microenvironments. (A) Schematic diagram of direct, 3D bioprinted, cell-laden bone matrix as a biomimetic model for a breast cancer metastasis study. (B) CAD model of the 3D matrix. (C) 3D surface plot of the bioprinted matrix. Scale bar: 200µm. (D–G) Scanning electron micrographs (cross-sectional view) of porous matrices: (D) 10% GelMA, (E) 10% GelMA + nHA, (F) 15% GelMA, and (G) 15% GelMA+nHA, respectively. Scale bar: 100µm. The inset images are photographs of the corresponding matrices. (H,I) Fluorescence micrographs of the 3D bioprinted MSC-laden 10% GelMA matrix; 3D bioprinted cells were prelabeled by Cell Tracker Green CMFDA dye. GelMA; gelatin methacrylate (A–I reproduced with permission from Zhou et al., 2016).
3D bioprinting to reconstitute brain cancer glioblastoma TME
Glioblastoma (GB) is the most prevalent and lethal adult primary central nervous system cancer with few treatments and poor prognostic.
The conventional preclinical drug development process relies on in vitro and in vivo studies. First, the drug toxicity/efficacy is tested in 2D cell culture, then, patient-derived xenografts are realized on mice, implanting a small piece of the tumor’s patient into immunodeficient mice.
In a study published in Science (4), the authors created a 3D bioprinted vascularized glioblastoma model using a polymeric bioink based on fibrinogen and gelatin to provide a 3D structure for GB cells and including a functional vascular network created using a sacrifice bioink, composed of Pluronic F127 and Thand then injected HUVEC cells and pericytes cells, after the bioink melts.
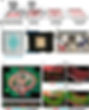
Figure 2. Fibrin brain-mimicking 3D-bioink integrated with 3D engineered printed perfusable vascular network.
(A) Schematic illustration of the 3D-bioprinting model multistage process. (B) 3D-printed Pluronic-based vascular bioink (in cyan) on top of 3D-printed layers of fibrin 3D GB-stroma bioink (in white). (C) 3D-bioprinted vascularized GB model sealed in a metal frame showing the complete perfusion chip. (D) The vascularized 3D-bioprinted GB model is connected to a peristaltic pump through a tubing system and placed in a designated incubator. (E) Tiled Z-stack confocal microscopy images of the 3D-printed penta-culture vascularized GB model. Blood vessels are lined with iRFP-labeled hPericytes (in cyan) together with mCherry-labeled HUVEC (in red) (107 cells/ml; 4:1 ratio) and surrounded by azurite-labeled PD-GB4 (in blue), GFP-labeled hAstro (in green), and nonlabeled hMG (2.1 × 106 cells/ml; 1:1:0.1 ratio). The dashed box represents a coronal cross-sectional plane of the vessel. (F) Fluorescence microscopy images of the 3D-bioprinted vascularized GB model before (top) and after (bottom) perfusion of 70-kDa dextran-FITC. The 3D-bioprinted model is composed of a fluorescently labeled vascular network (mCherry-labeled HUVEC and iRFP-labeled hPericytes) surrounded by nonlabeled GB-bioink (hAstro, PD-GB4, and hMG).
The results of the study showed that the 3D bioprinted glioblastoma cells formed tumors that were similar to those seen in human patients, resuming the principal characteristic: volume, growth kinetics, invasion capabilities, response to therapies, and their genetic signatures; Recent studies have suggested that astrocytes play a crucial role in shaping the TME and may contribute to treatment resistance in brain tumors. Then, they incorporated astrocytes and microglia into this 3D GB model and observed that astrocytes enhanced GB aggressiveness. (4)
Many cancer models have already been described (5). These bioprinted cancer models are up-and-coming platforms for evaluating personalized drug screening of anti-cancer drugs as they seem to reflect the native tumor truly. They can improve the biological and physiological relevance of in vitro cancer models.
And what about you? Did you know you could now start your own cancer model using TissueRay and GelMa bioink? If you get interested, contact our support team; they will gladly help you!