3D bioprinting is an innovative technology that holds great promise for improving the future of medicine. It allows the construction of 3D tissue or organ structures by depositing biomaterials together with cells - the bioink - using a computer-aided printer. This technology is already used as an in vitro tool in basic research and for drug testing and, in the future, it might also help to overcome the lack of organs for transplant.
There are three main types of 3D bioprinting: light-based bioprinting, inkjet/droplet-based bioprinting, and extrusion-based bioprinting. In all of these methods, the bioink is an essential element. Its deposition is synchronized with the motorized stage movement and crosslinked simultaneously or just after the printing to stabilize the product (1).
The success of the printing depends on the quality of the bioink and selecting the most appropriate one can be challenging. The biomaterial must exhibit physical, physicochemical, and mechanical properties that are compatible with the bioprinting process and with the cells. Therefore, we must consider characteristics such as the chemical composition, crosslinking density, gelation process, rheology, biocompatibility, and biodegradability (Fig.1) (1,2,4).
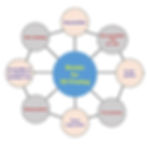
Fig.1: Important requirements for selecting a bioink for 3D printing in biomaterial aspects (4)
The bioinks are constituted from hydrogels - crosslinked hydrophilic polymer chains that can not dissolve in water - which can be either from natural or synthetic origin.
Most natural bioinks can mimic really well the extracellular microenvironment (ECM). They can be originated from:
- Polysaccharides, where alginate is the most represented
- Hyaluronic acid
- Proteins: collagens, gelatin, fibrin, elastin, etc.
- Decellularized extracellular matrix: collagen, glycosaminoglycan, etc. (4)
The most commonly used synthetic polymers are pluronic and polyethylene glycol (PEG) (4).
Natural hydrogels provide the desired microenvironment for cell attachment and proliferation, since they can mimic the native ECM, however, their physical and/or mechanical properties are sometimes not ideal for building complex structures. Therefore, it is common to combine them either with another natural polymer and synthetic polymers, or even perform chemical modifications to get more stable structures. GelMA (gelatin methacrylate) is a good example of a natural-derived bioink that has been chemically modified to be used in stereolithography bioprinting (light-based bioprinting). The methacrylate groups introduced in its structure allow the obtainment of covalent bonds between polymer chains (under appropriate light irradiation), which are stronger than only physical interactions of thermally crosslinked gelatin, improving the mechanical strength of the material. (1)
Rheology is also a crucial parameter when formulating a bioink. For instance, in extrusion-based bioprinting, bioinks with shear-thinning behavior are desirable to avoid a decrease in cell viability. It means that the material’s viscosity decreases as shear stress increases, which allows the bioink to pass easily through the extruding needle avoiding high shear stress on cells (4). At the same time, the material must harden quickly after being extruded to maintain the desired shape of the printed structure. If a bioink can’t succeed in maintaining the structure, it might require the use of other bioinks, such as a support bioink, which helps to maintain the final structure, or a sacrificial bioink, allowing the printing of more complex structures that can easily be removed after the printing. (2)
The gelation of the bioink is obtained by crosslinking the polymer chains. It can occur either by a physical/reversible or a chemical/non-reversible process (Fig.2). Physical crosslinking includes thermocondensation, self-assembly, ionic gelation, or electrostatic interaction, which generally depends on the intrinsic properties of the polymers. This type of crosslinking limits the ability to fine-tune the attributes of hydrogels but is easy to achieve. Chemical crosslinking, on the other hand, is achieved by covalent bonds. It is a more precise process but irreversible. The great advantage is that the mechanical properties of chemically crosslinked bioinks are superior to that of physically crosslinked materials.
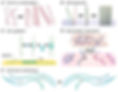
Fig2: Crosslinking of hydrogels (5)
(A to D) Physical crosslinking. (A) Thermally-induced entanglement of polymer chains. (B) Molecular self-assembly. (C) Ionic gelation. (D) Electrostatic interaction. (E) Chemical crosslinking.
Summing up, the choice of the bioink is crucial in the bioprinting process. It must be compatible with the bioprinting process and present adequate properties to promote cell attachment and proliferation. Some bioink properties should receive particular attention: viscosity, gelation process and biocompatibility. The combination of different types of bioinks or the chemical modification of their structure seems to be a promising way to optimize bioprinting efficiency.
At TissueLabs, we offer the MatriXpec™ hydrogels, obtained from decellularized ECM and available for fifteen different types of tissues or organs. Also, we offer MatriXpec™ GelMA, which can be covalently crosslinked under UV light irradiation, so you can develop your ideal experiment.
References:
(1) Bioinks for 3D bioprinting: an overview. P Selcan Gungor-Ozkerim Biomater Sci. (2018).
(3) Candidate Bioinks for Extrusion 3D Bioprinting—A Systematic Review of the Literature.
Tarassoli S. et al, Front. Bioeng. Biotechnol., (2021)
(4) Recent trends in bioinks for 3D printing. Gopinatha J et al., Biomaterials Research Volume 22, Article number: 11 (2018)
(5) Advances in engineering hydrogels. Shrike Zhang Y. and Khademhosseini A. Science. 2017.
(6) Bioinks and bioprinting technologies to make heterogeneous and biomimetic tissue constructs Ashammakhi N. et al., Mater Today Bio. (2019)